- Date
- Friday, April 7, 2023
- Author
- Ryan Buhler, Forecast Program Manager
The deep persistent problem has dominated our messaging this season and has been the suspected avalanche problem in ten (83%) of this season’s fatalities. As we near the end of this unusual winter, let’s look back at the weather and snowpack conditions that formed this problem and kept it firmly in our concerns.
A brief summary
- Snowpack faceting creates weak snow grains. It occurs with extended periods of cold temperatures and in shallow snowpacks.
- We had below average snow depth and several periods of cold weather during November and most of December, which led to the development of a major weak layer at the bottom of the snowpack.
- In the southern Kootenays, most of the South Coast, and parts of the NW Coast, a rain crust formed in December. This crust formed above the weak layer at the base of the snowpack which substantially reduced the likelihood of human triggering of the deep weak layer.
- As the snowpack grew and went from significantly below average to average in February, exceeding 2 m in depth, deep persistent slab activity became less common in many of the deep snowpack areas through the Columbia Mountains. The deeper snow meant the layer was too deep for a human to directly trigger.
- The problem continues to remain a major concern in shallow snowpack areas, especially in the Purcells and Central Rockies.
- When the winter snowpack transitions to a spring snowpack, any bridging we observed from the rain crust and the effects of the deeper snowpack may be negated. As we move into spring, deep avalanches are possible in all regions—even those where the problem hasn’t been a major concern this winter.
For background information, check out the following blog posts:
- Dec 15, 2022: Persistent to Deep Persistent – Why the Switch?
- Jan 5, 2023: The Persisting Problem
- Jan 27, 2023: Deep Persistent Slab Management and Mindset
- Mar 2, 2023: The Deep Persistent Slab Problem is Not Going Away
Snowpack Faceting
Before we get into the weather and snowpack data, it is helpful to review the process of snowpack faceting. Faceting is a snowpack metamorphism process by which the snow grains transform into larger, angular grains. Because these larger, angular grains have less surface contact with the adjacent grains, they are considered a type of weak snow crystal and commonly create persistent weak layers in the snowpack. Cold temperatures promote this faceting process. Facets are often described as sugar-like because they are loose and you can sift the snow through your hands like sugar. If you’ve ever tried to make a snowball in a cold place with a shallow snowpack and the snow won’t stick together no matter how hard you try, that is faceted snow.
Snowpack faceting occurs when the snowpack is subjected to a strong temperature gradient for an extended period of time. Because the ground below the snowpack is effectively always at or near 0 °C, the temperature gradient is determined by the air temperature and the snowpack depth. The general threshold for temperature gradients is 10 °C per 1 m snow depth. If the gradient is greater than this, we can expect faceting, and if it is less, we can expect rounding.
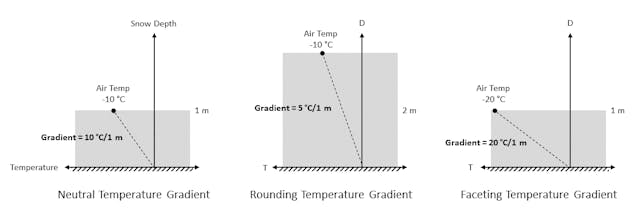
Simplified temperature gradients in the snowpack showing how rounding and faceting occur.
There are two basic conditions that typically lead to prominent facet growth in the snowpack: extended periods of cold temperatures and shallow snowpack depths. When both of these conditions exist, we can expect the worst case scenario for facet development. And this is basically what happened through the early winter season for much of the interior.
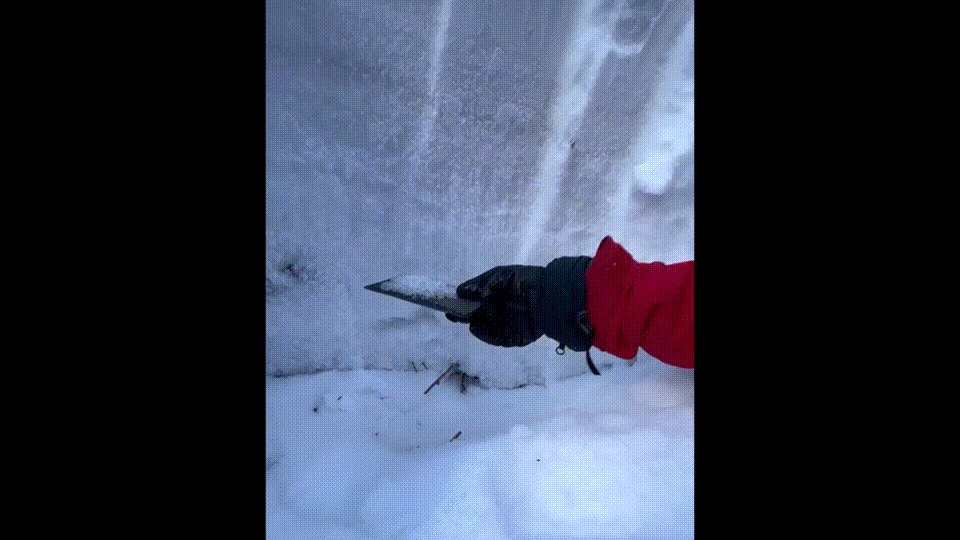
An example of the weak facets at the bottom of the snowpack in the southern Rockies
Early Season Weather and Snowpack Data
Glacier National Park keeps manual height of snow and temperature data from the Mt. Fidelity weather station which sits at treeline elevation (1905 m) and is updated daily. This is highly reliable data that dates back to the early 1960s, so it is useful for research and reviewing historical conditions. It is important to note that this is only a single location and conditions will vary throughout the interior. However, it provides a baseline for looking at our early season conditions in the interior and comparing them to historical values.
Mt. Fidelity manual height of snow, air temperature, and precipitation data for September 2022 to February 2023 provided by Parks Canada.
October and Early November
One of the most memorable things from last fall was how warm and dry it was, and how late snowfall arrived in the interior mountains. On the Thanksgiving long weekend, it was still hiking season in the alpine—whereas in normal years, many keeners are already finding pockets of snow to ski by mid-October.
When snow finally arrived in late October, it came in heavy and we saw almost 1 m of snow accumulate by November 6. This is the snow that evolved into the deep persistent weak layer.
Mid-November
The next two weeks (Nov 7-Nov 21) were dry and relatively cold. This was the initial period of faceting and weak layer formation. In addition to the extended dry period, we also saw strong northerly outflow winds for much of this time which redistributed the snow in any exposed alpine terrain. There was also one notable warm-up in the middle of this period with an inversion and some alpine areas saw above freezing temperatures for a brief period on November 15. In wind-sheltered areas at lower elevations, we also observed the growth of large surface hoar. Steep south-facing slopes saw the formation of a sun crust during this period.
Just before this complicated early season snowpack was buried, it was described as having the following characteristics:
- Around 1 m snow depth in wind-sheltered terrain at treeline in the deepest snowpack areas of the Columbia Mountains.
- Around 50 cm average snow depth in wind-sheltered terrain at treeline in the Purcells and Rocky Mountains.
- In the alpine, highly variable snow depth (30-80 cm) due to wind stripping and redistribution from a prolonged period of northerly outflow winds during the drought.
- In the alpine, hard wind slabs were faceting out with persistent cold temps.
- In deeper snowpack areas, the upper snowpack was composed of up to 30 cm of facets.
- In shallower areas, the entire snowpack was generally faceted throughout.
- Sun crust had formed on steep solar aspects, up to 2 cm thick.
- A brief period of warming and temperature inversion during the drought may have contributed to surface crust formation in some alpine areas.
- Widespread surface hoar up to 50 mm could be found in wind-sheltered terrain.
These are just a selection of observations to highlight the general snowpack conditions prior to burial, but conditions likely varied across the interior.
Following this two-week period of weak layer formation, another 50+ cm accumulated in the last week of November, burying the layer.
December
Because snowpack faceting occurs in shallow snowpacks during cold periods, it is normal to expect a layer of facets at the base of the snowpack in many seasons. But they don’t always lead to a prominent persistent weak layer, especially one that lasts for most of the season. In many seasons, the facets at the bottom of the snowpack get compacted below the ground roughness if the facet layer is thin or from the weight of heavy snow accumulations through December, may undergo rounding with mild temperatures and deeper snowpacks, or get capped by a firm melt-freeze crust. I expect if we had a normal mild and snowy December, we wouldn’t still be discussing this layer in March.
December was notable for being much colder and drier than is typical. In the Mt Fidelity dataset, we saw two periods of Arctic air. The first occurred at the beginning of December and saw cold and dry conditions for around eight days. The second occurred just before Christmas and saw temperatures reach near -30 °C for around five days. During both of these periods, the snowpack in the relatively deep snowpack area of Mt Fidelity was only slightly above 1 m and in many other areas, such as the Purcells and Rockies, the snowpack was still only in the range of 50-70 cm. This means the snowpack was continually faceting through both of these periods and further weakening the already weak snow from November at the base of the snowpack.
A major storm occurred at the end of December, which formed a substantial storm slab above the weak snowpack from November and early December. We began to see large avalanches occurring on the deep layer during this period and issued a Special Public Avalanche Warning on December 28 that warned about the dangerous conditions caused by this deep layer.
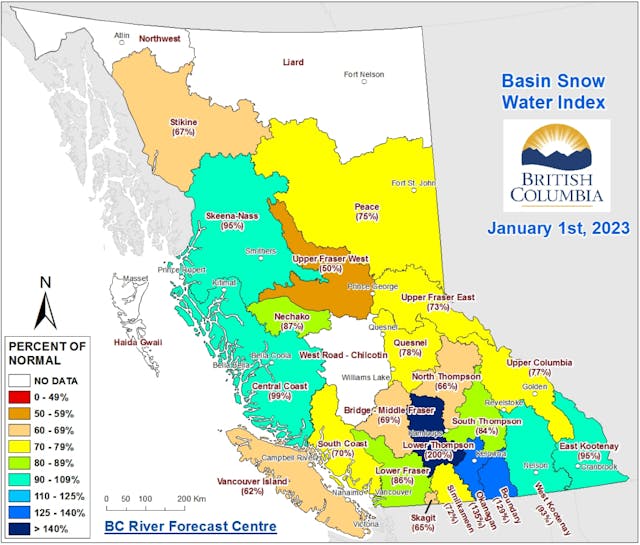
Even after the major storm at the end of December, the snowpack throughout the interior was still well below average for many parts of the interior at the end of the month. Through most of December, the snowpack throughout the interior was very shallow compared to average. Source: BC River Forecast Centre
Regional Analysis
The Mt Fidelity data is very useful for comparing to historical data, but it is also important to look at regional trends. Several other points were selected, including one in each of the Purcells, Cariboos, southern Kootenays, and Rockies.
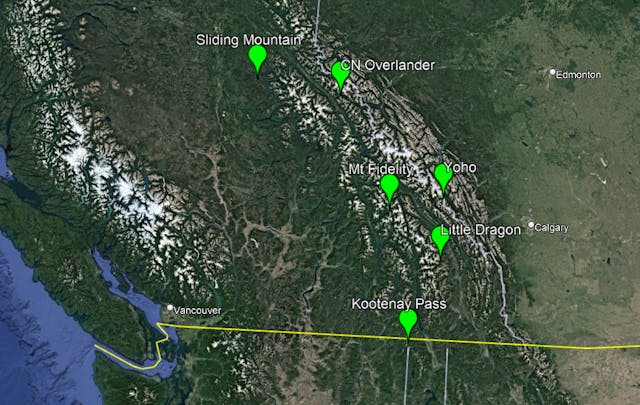
Location of weather stations for analysis.
This data is presented below. It is not compared against historical values like Mt Fidelity, but rather compared against each other. Given that these stations are in a variety of locations and elevations throughout the interior, they show surprisingly similar temperatures—to the point where it is difficult to differentiate between the lines in the graph. We can interpret this to mean the cold temperatures described at Mt. Fidelity occurred throughout the interior.
The snow height data deviates more than the temperature data, but the trends are the same. Mt. Fidelity is the deepest snowpack of the six stations analysed, so if we are assuming substantial faceting occurred at Fidelity, we can also assume it also occurred in the rest of these locations. In fact, we would expect that the faceting was actually more substantial in the shallower snowpack areas. This snowpack data might be a bit misleading with several of these stations being lower elevation than ideal. The deep persistent problem seems most problematic at upper treeline and alpine elevations, but good snow height data is often not available at these higher elevations, so we use lower stations as a proxy. For the few snow height lines at the bottom of the graph, we might assume the representative snow heights are a bit deeper than presented on the chart below.
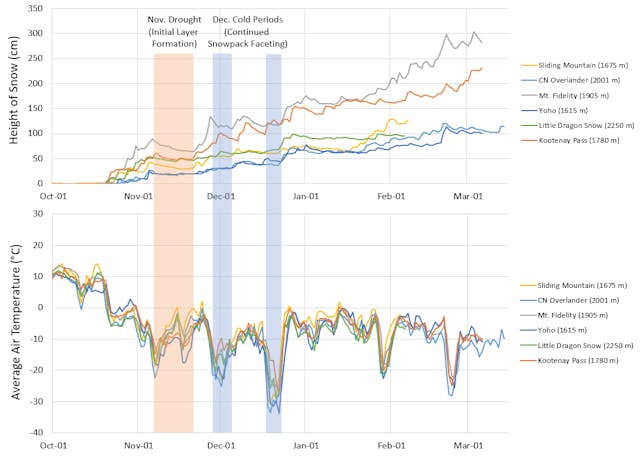
Average air temperature and height of snow data for six stations in interior BC.
Snowpack Bridging
A discussion of deep persistent problems wouldn’t be complete without including the concept of snowpack bridging. An astute reader might be asking about Kootenay Pass and the general lack of a deep persistent problem in that region, despite the data above showing the same general conditions during the early season. The concept of bridging is that strong layers of snow above a weak layer can prevent the stress of a human from reaching and triggering a weak layer. This concept comes into play this season in two ways: melt-freeze crust bridging and deep snowpack bridging. In the case of Kootenay Pass, a melt-freeze crust bridging the weak layer is thought to be the main reason for the lack of a deep persistent problem.
Melt-Freeze Crust Bridging
The first concept of bridging is generally related to melt-freeze crusts but can apply to other firm, dense layers as well. Basically, if a crust is thick and/or firm enough, it can prevent the stress of a human from penetrating deeper into the snowpack. This is common with thick or icy rain crusts. Generally speaking, you can imagine that if a crust is thick and/or firm enough to support the weight of a human when the crust is on the surface of the snow, it is also going to be able to prevent the stress of a human from penetrating deeper into the snowpack and causing failures on deeply buried layers once it is buried. This is often referred to as a capping crust because it is creating a cap on the snowpack making the human triggering of any deeper weak layers much less likely.
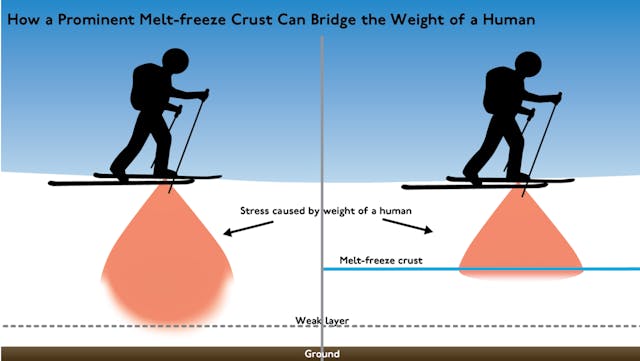
A firm melt-freeze crust can prevent the stress of a human from reaching deeper into the snowpack. This is often referred to as a capping crust.
While it isn’t immediately apparent in the data presented above, in late December a period of rain during a big storm around Christmas impacted the north and south coast, and the southern Kootenays near the US border. This rain crust was strong enough to create a capping layer above the basal weakness and has generally prevented the November weak layer from creating a deep persistent slab problem in these regions. This is illustrated in the map below, where areas with a capping layer are excluded from the regions of most concern.
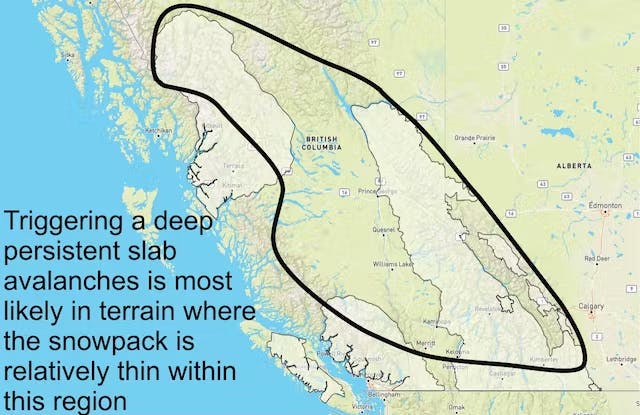
This map was published on March 2 in the blog post called “The Deep Persistent Slab Problem is Not Going Away” and excludes areas of the southern Kootenays, South Coast, and Northwest Coast because of rain crust, which formed above the deep weak layer and is effectively capping the layer. Source: The Deep Persistent Slab Problem is Not Going Away
Deep Snowpack Bridging
The second important concept related to bridging is deep snowpack bridging. The typical maximum stress of a human travelling under normal conditions, whether it is a skier or snowmobiler, is around 1 m or so in depth. This does not include the component of snowpack penetration, so if your skis or snowmobile track are penetrating 50 cm into the snowpack, it is possible to trigger a layer around 150 cm down. Of course, this depends on many factors, such as snowpack conditions, but provides a rough idea of how deep a layer needs to be before it becomes unlikely that it would be triggered directly by a human. Although extra stress caused by jumping or crashing might make it possible to trigger something a bit deeper.
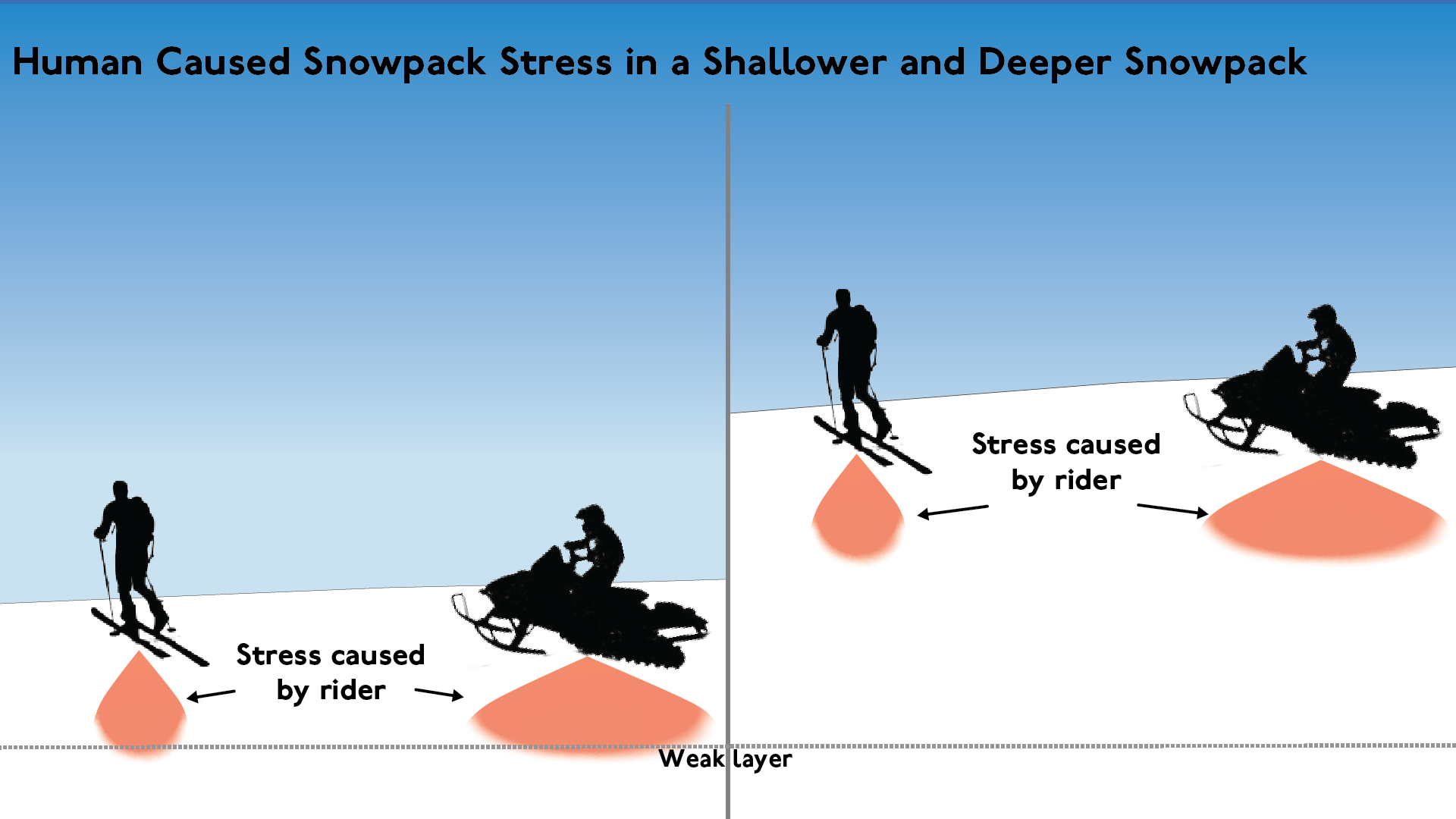
With a deeper snowpack, the middle of the snowpack can create a bridging effect, making it difficult for the weight of a human to penetrate deep enough to trigger deep layers.
We saw a trend across regions related to the snowpack depth. The first round of avalanche activity on the layer occurred in deeper snowpack areas in the Columbia Mountains because it was the first area to form a thick enough slab for avalanches to happen. It took longer for slabs to develop in shallower snowpack areas of the Purcells and Rockies and consequently it took longer for the deep persistent problem to develop as well. Later in the season, the deep snowpack areas were the first to see the problem become less severe, while the problem persisted in the shallower snowpack regions.
February was a snowy month and we saw the snowpack go from well below average to return to average levels. As a result, the deep layer was now more than 2 m deep in the Columbia Mountains, as seen in the Mt. Fidelity data. Once it was buried this deep, it became difficult for a human to trigger the layer directly in most areas and we saw avalanche activity taper. By the end of February, the layer was down almost 3 m and direct triggering became quite unlikely. Of course, there are caveats to this. Areas with a thinner snowpack, particularly rocky alpine regions where strong winds prevent snow accumulation, remain a concern. Caution should still be exercised when venturing into these areas.
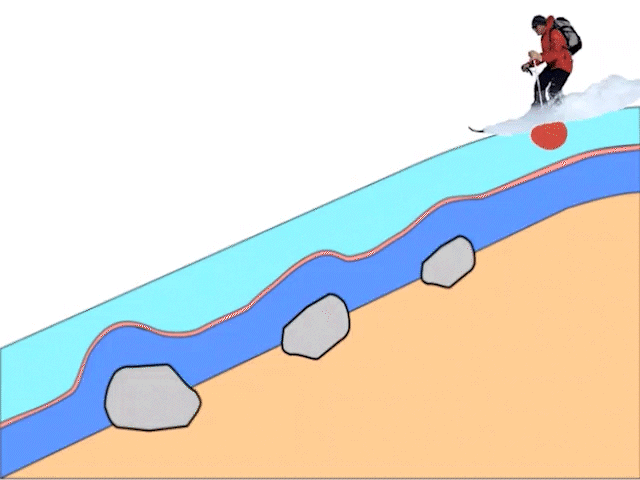
This excellent animation shows the process of a skier triggering a weak layer and also highlights thin spot triggering. It is important to remember that even in a region with a deep snowpack, it may still be possible to trigger a deep layer in an area where the snowpack is shallow. Source: Bruce Jamieson, An inside look at how people trigger slab avalanches
Late Spring and Isothermal Snowpack Conditions
An isothermal snowpack means that the snowpack is the same temperature from the surface to the ground, 0 °C throughout. When this occurs, any bridging that we might have been relying on goes out the window and full-depth avalanches become possible again—even in the regions like the South Coast and southern Kootenays where the layer hasn’t been a problem for most of the season. We normally expect the alpine snowpack in the Columbia Mountains to become isothermal in May, but it is possible that it could happen sooner with a hot April or later if we have a cold spring.
While the snowpack going fully isothermal is expected to result in the layer waking up in many areas, it is likely that the layer will also wake up in some areas sooner when the winter snowpack in the alpine starts to transition into a spring snowpack. Some of the conditions we are watching for include the following:
- Freezing levels reaching mountaintop elevations coupled with strong solar radiation
- Sustained high freezing levels for multiple days, especially if temperatures remain warm overnight
- A substantial alpine rain event
These types of warming will likely start to wake up the problem in shallow snowpack areas first. As more heat gets added to the snowpack, the problem will likely wake up in the deeper snowpack areas. When the snowpack finally goes fully isothermal in late spring, all bets are off and very large, full depth avalanches become possible again everywhere, even in regions where the layer hasn’t been a problem for most of the season.
Summary
This is a long blog that came together over several weeks of digging into early season data and reflecting on a season dominated by the deep persistent slab problem for many of our regions. We had the perfect conditions of a low snowpack in November and most of December, combined with mainly cold temperatures during the same period, and cold temperatures through most of January and February. As a result, we had prime conditions for the weak layer to form at the bottom of the snowpack and very limited melt-freeze crust formation through the middle of the winter, meaning no strong bridging layer to cap the deep weak layer. The Kootenays and coastal areas remained an exception as a capping rain crust significantly reduced the likelihood of humans triggering the deep persistent problem.
We now have a deep snowpack through most of the interior and likely have a new surface crust from the recent sunny weather (although it’s likely that this crust does not exist on north aspects) which has given us a break from the problem in many areas. The expectation remains that shallow snowpack areas, especially in the Purcells and Central Rockies, may still be places where it is possible for a human to trigger the deep persistent layer directly. And as we head into spring, it is important to remember that cornice falls and avalanches in motion can trigger the layer. Extra caution is warranted during the heat of the afternoon when the snowpack will be the least stable. Finally, when the snowpack becomes isothermal or near isothermal in the late spring, all bets are off and we expect the snowpack bridging to break down. When this happens, we will likely see another round of full-depth avalanches occurring naturally in all regions.